Shifting our ideas about… Weight Loss (perspective article)
Reducing calorie intake may not help you lose body weight – true or false? TRUE!
Weight-Centred Health Paradigm (WCHP)
It’s a good idea to be constantly checking what we think we know to be true. Asking questions about nutrition science, for example, and what it means for our health. Such as the weight-centered health paradigm (WCHP) underlying the weight-normative approach. This paradigm places emphasis on weight and weight loss when defining health and well-being, and it conveys weight management in terms of a calorie-centric model. By calorie, we mean the energy value of foods calculated in caloric values.
In popular health, the calorie-centric model is synonymous with ‘calorie counting’, a euphemism for weight-watching, where calories are often erroneously described as good or bad (depending on nutritional value) and body ideal appearance is often conflated with good health. The national dietary guidelines endorse ‘eat less move more’ and the notion of willpower is regularly discussed in the same context. It’s a one-size-fits-all approach, where a calorie is a calorie regardless of individual differences in the population.
Ultimately, maintaining a healthy weight requires regulation of energy balance between nutritional energy intake and energy expenditure. A fundamental principle of metabolism and nutrition is that body weight change is an imbalance between energy intake (content of food eaten) and energy expenditure (using energy to live and work).
Figure 1 depicts this as a schematic representation, where Ein is nutritional energy intake and Eout is nutritional energy expenditure. The equation implies that weight gain can occur when the body’s energy balance is positive and weight loss when the body’s energy balance is negative. But is it really that simple?
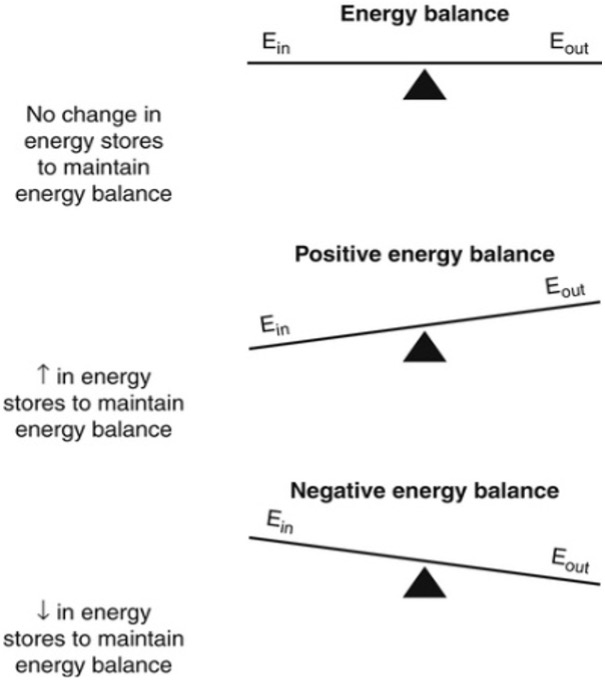
Snapshot of Whole-body Homeostasis
Whole-body energy homeostasis refers to the self-regulatory mechanism through which our internal equilibrium is preserved while adapting to fluctuations in the external environment.
Key components maintaining internal equilibrium:
Energy intake: regulation of food-seeking, consumption, satiety and nutrient absorption;
Energy expenditure: control of metabolic processes that utilise energy, including basal metabolic rate, physical activity and thermogenesis or temperature regulation;
Hormonal regulation: hormones such as insulin, leptin and others play essential roles in signalling energy status to the brain and peripheral tissues about hunger and satiety for example;
Metabolic set-point: the establishment of a metabolic “set-point” or a theoretical baseline for energy balance that the body seeks to maintain;
Neural circuitry: in the brain orchestrates responses to sustain energy balance. This includes regulating essential functions like respiration and heart rate while ensuring the metabolic needs of all cells are met.
Key components in the external environment include:
Environmental Cues: External factors like the availability and types of food in the environment, influence food-seeking behaviours and satiety.
Climate: External temperature variations can affect energy expenditure, including thermogenesis.
Physical Environment: The surroundings and lifestyle choices in the external environment can impact energy expenditure through physical activity, for example.
Whole-body energy homeostasis is contingent upon the dynamic communication between the central nervous system (CNS) brain and spinal cord relaying information to the rest of the body, This neural communication enables the transmission of electrical impulses and the exchange of neurotransmitters..
Circuits within the central nervous system (CNS) consistently assess and integrate these signals from metabolic, hormonal, and neuronal sources. This complex dialogue among cells and organs governs metabolic processes, overseeing the storage and utilisation of nutrients (energy balance) and adjusting eating behaviours to align with the body’s energy demand.
Different brain regions and hormones, collaborate to finely regulate various aspects of food seeking, consumption, and satiation. This continual fine-tuning of homeostatic controls ensures optimal conditions for survival. The energy homeostasis principle is effectively illustrated in Figure 4 schematic:

Energy Homeostasis
Energy homeostasis is the ‘regulation of daily total nutritional energy intake and expenditure in the maintenance and defence of energy balance’. It is a potentially powerful tool for investigating weight management science. Weight stability is achieved only by bringing into play adjustments in energy intake and/or energy expenditure appropriate to compensate for threats to that stability.
How do our cells obtain energy to sustain energy homeostasis? Nutritional energy can be categorised as “metabolic fuels” derived from fats (lipids), carbohydrates (sugars) and proteins. These macronutrients have differing energy densities related to their proportions of carbon, hydrogen and oxygen atoms with a metabolic equivalent of calories (fats approximately 9kcal/g and proteins & carbohydrates 4kcal/g).
Inside cells, energy is supplied through the oxidation or burning of these metabolic fuels through a process called oxidative phosphorylation. This process takes place within mitochondria in the cell. Mitochondria are often referred to as the “powerhouses of the cell” because they are the primary sites for ATP (adenosine triphosphate) synthesis produced through oxidative phosphorylation.
Mitochondria are involved in both the production and breakdown of ATP, fundamental to active metabolism. Some of the other critical cellular processes that rely on the energy released from ATP breakdown include DNA replication, RNA synthesis and cell division.
Basal Metabolic Rate and Resting Metabolic Rate
Two key parameters in determining energy balance are Basal metabolic rate (BMR) and resting metabolic rate (RMR). The two are often used synonymously, referring to the rate of oxygen uptake, with a metabolic equivalent of calories burned or energy expended for basic functions. RMR is while at rest (without any physical activity) and BMR is while at rest and fasted (calculated 12-18 hours following the last meal).
BMR makes up 60 -70% of the energy expended or oxygen consumption. Diet-induced thermogenesis or energy expended after a meal is 10 -15%, and thermogenesis or body temperature regulation and physical activity is 10 -15%. Postural maintenance and even fidgeting account for 6-10% of the energy expended. BMR has been reported as proportional to body mass. This means basal oxygen consumption tends to be higher in men than in women, and relative to body mass index or BMI.
BMR increases as body weight increases and decreases as body weight decreases as we might expect. So, energy expended (the equivalent of calories burned per kilogram of body weight), decreases as our body weight decreases, as dietary reductions result in metabolic inefficiencies. And despite having higher total daily energy expenditure levels as body weight increases, energy expended is lower in relation to body weight.
What’s Going On?
This creates the ideal situation in either case for weight gain and regain in the body’s attempts to maintain homeostatic control of energy balance. People frequently describe instances where they often “sabotage” or derail their diet efforts. Similarly, studies have highlighted “constant weight fluctuations” or “repeated cycles of weight loss and regain” (1994) or “rapid weight loss followed by a plateau and gradual regain” (2018). The brain is constantly managing homeostatic controls to safeguard against energy imbalance.
A high BMR indicates increased overall body metabolism, meaning more calories are burned to sustain you throughout the day. Conversely having a low BMR indicates reduced overall body metabolism, and is an independent predictor of future weight gain.
This poses a risk characterised by heightened immune and pro-inflammatory responses. Referred to as low-grade systemic inflammation, this chronic response, often associated with diseases like obesity and COVID-19, entails sustained, low-level inflammation throughout the body.
Persistent inflammation can impact not only fat cells but also the functioning of the immune, endocrine (hormone), and other bodily systems, including the heart. These effects are linked to disrupted energy homeostasis, revealing unexpected consequences of chronic inflammation associated with WCHP.
The fundamental principles of whole-body energy homeostasis offer insights into the intricate mechanisms governing weight regulation. Notably, dispelling the efficacy of WCHP and the notion of calorie counting as a standalone approach to weight management. This discussion aims to dispel and turn attention to a Metabolic-Centred Health Paradigm (MCHP) that centres on the foundational principles of whole-body energy homeostasis. Reflecting a nuanced understanding of the multifaceted nature of energy balance and its role in weight management.
INTERLUDE: Macronutrients in Health and Disease
Dietary carbohydrates (simple sugars or complex starches) are metabolised into glucose in the body. Glucose is one of the main energy sources for cellular metabolism. We know that our cells on uptake of glucose can produce ATP that is critical for metabolic and cellular processes (transfer of energy to cells).
Insulin has several functions, including stimulating the uptake of glucose, amino acids, and fatty acids into muscle, liver and fat cells. It promotes the storage of these substances in the form of proteins in muscle, glycogen in the liver and fat in fat cells.
Monitoring blood sugar levels during and following a meal reveals that certain foods can cause significant blood sugar spikes and if persistent can lead to a decreased ability of the body to effectively lower blood sugar levels. This situation can result in hyperinsulinemia, which means there is an excess of insulin in the body. Hyperinsulinemia, in turn, can contribute to the development of insulin resistance.
Insulin resistance, a key factor of low-grade systemic inflammation, is characteristic of metabolic conditions like type II diabetes and metabolic syndrome. This manifests as persistent feelings of hunger, difficulty achieving satiety, elevated blood pressure, increased abdominal obesity, and a lowered BMR. Substantial research documents a positive relationship between elevated insulin levels in the bloodstream and obesity.
Insulin resistance presents a hurdle in weight loss efforts. Not least insulin promotes the storage of fat in fat cells, heightened insulin levels impede the breakdown of fat, causing an enlargement of fat cells. So, managing blood sugar spikes through dietary choices, plenty of sleep, hydration and movement can influence how fat cells respond and weight management.
Blood sugar regulation is significantly influenced by age, health, and lifestyle factors. Conditions such as menopause, dehydration, low salt intake and a sedentary lifestyle can detrimentally impact blood sugar control. Similarly, diets rich in simple sugars as well as saturated fat have pro-inflammatory effects that may negatively affect blood sugar responses, the extent is unique to each individual.
Dietary fat and oil or triglycerides are converted to fatty acids and glycerol and stored in adipocytes (lipids or fat cells). Lipolysis is the process of mobilising this stored energy to fuel the body and for insulation, temperature control, and other critical functions for life. Blood glucose homeostasis is closely linked with our fat cells. It is the glycerol component of the triglyceride, that gets readily converted into sugar or glucose for use as energy by the body.
Dietary ‘unsaturated‘ fats, such as olive oil, avocado, nuts and seeds, have been shown to support insulin regulation and the biological action of insulin on our fat cells. This is contrary to popular health low-fat regimens which don’t tend to differentiate between saturated and unsaturated fats. We know that prolonged exposure to excess dietary ‘saturated’ fat can elevate fatty acids circulating in the bloodstream, implicating cholesterol levels.
Proteins in food are used to make amino acids that in turn make proteins used in the body. Serving as the backbone for compounds like our hormones and neurotransmitters in the brain. Just 20 amino acids make many thousands of proteins in the human body, required for neural and cell function, repair and regeneration, so they are critical. Some proteins are made in the body and some are essential amino acids or must be supplied by the food we eat.
Dopamine, for instance, is a derivative of the amino acid phenylalanine (which is an essential amino acid) and also tyrosine. High phenylalanine foods include meat and poultry, fish, tofu, beans, milk, nuts, seeds, pasta, whole grains, and vegetables like sweet potatoes. High-tyrosine foods are sesame seeds, cheese, soybeans, turkey, beef, pork, fish, eggs, nuts and legumes.
Serotonin is synthesised from the essential amino acid tryptophan. Tryptophan can be found in nuts, seeds, dairy, red meat, poultry, pork, fish, oats, beans, lentils, eggs, dark chocolate, peanut butter, pumpkin seeds, sesame seeds, sunflower seeds, soybeans, tofu, soy milk, spirulina and wheat flour. See here for guidelines on how much is required by kg of body weight.
Any excess protein is turned into glucose via glucogenesis in the liver (by a hormone called glucagon). Any excess glucagon with the help of insulin is converted into fatty acids and stored as fat and glycogen in the liver. Glycogen reserves are used to prevent low blood sugar when we are sleeping or in a fasted state.
Insulin resistance in muscle tissue and non-alcoholic fatty liver disease are linked to dysregulation of glycogen metabolism which alters glucose metabolism.
Evaluating Some of the Evidence for a Shift in Paradigm
On paper, the WCHP suggests that we will lose weight if we make relatively consistent negative energy changes (caloric deficit) over time, alongside some kind of aerobic physical activity. To quantify this, the caloric equivalent of losing one pound of body fat is 3750 calories. This applies the first law of thermodynamics to human metabolism, which assumes:
1) We are a perfect thermodynamic machine (i.e., a closed system able to capture and qualify and account for every unit of energy); and
2) That the metabolic value of calories from different fuels (fat, carbohydrates and protein) behave the same metabolically in different people.
However, humans are an open system. Plus, we know that no two people’s responses to individual foods are the same, even between identical twins as shown in twin studies.
A Professor of bioenergetics in the US, Ben Bikman suggested an alternative ‘endocrine centric paradigm‘ to WCHP, which directs attention to the relevance of hormonal interactions with fat cells, chemically instructing the cells what to do with energy. His latest research showcases this:
“In a petri dish I can have ample calories from glucose and fatty acids or fat, and the fat cells won’t grow at all…, until we spike insulin into the culture. At the moment we add insulin … we can start to detect fat droplets … and over time these become big bubbly fat cells. This cannot happen unless insulin is elevated.
So a fat cell needs to be told by the hormone insulin, what to do with energy”. We know that pro-inflammatory foods can spike or elevate insulin. However, we also know there is high variability in individuals’ blood fat responses as well as blood glucose responses and inflammatory responses in response to different foods.
What Alternatives?
So, it’s not just about calorie counting, but food quality, as well as what and when we eat, how often, and even food combinations to keep the blood sugar spike down at each meal.
What about genetic factors at play in weight management? We know that people carrying two copies of the FTO gene (associated with a high obesity risk variant) are on average heavier than those carrying two copies of the protective version (associated with a low obesity risk variant). This absolute risk runs alongside a genetic susceptibility to obesity, whereby ongoing studies show human obesity can be inherited as a ‘polygenic trait’. This means many genetic mutations cumulatively might increase the risk of obesity ‘switched on’ by environmental factors, including lifestyle, and obesogenic environments. As well as social, economic and cultural factors.
Another study researching genetic susceptibility looked across a diverse population at multiple gene-nutrient interactions considering environmental factors and found gene mutations associated with a predisposition to obesity. This may explain the so-called obesity epidemic.
In addition, new genomic studies have discovered genetic traits behind disordered eating, and also variability in gut microbial composition as potentially having a heritable influence on weight management. So, weight management is far more complex than WCHP conveys.
Evaluating Further Evidence for a Shift in Paradigm
The percentage increase of age-specific population obese predicted in the U.K. in 2050 as compared with 2007 can be said to inform further the evidence of WCHP failure (see Figure 2). These results were modelled from a microsimulation using Department of Health obesity data and measures, on behalf of the U.K. government-initiated Foresight Programme. The results show correlative growth in obesity across the adult age groups, and combined three times percentage growth in the 1 to 20 age groups (2007, 17).
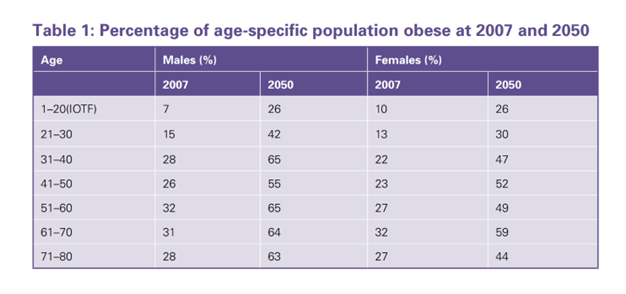
Figure 2: Govt Office for Science (2007, 17) Foresight Programme
That same Foresight Report suggested that “we are on average consuming too many calories on a regular basis. Latterly, data gathered by Public Health England (PHE) in 2017 overlaid the Foresight observations, with findings that obesity prevalence in the U.K. adult population between 1993 and 2019 has indeed grown. Increasing from 27% to 64%’ over that period (2017). While roughly 1.4 million children aged between 2 and 15 were classified as obese in 2018, with obesity rates for older children (10- to 11-year-old’s) increasing from 18.7% to 20.2% between 2009/10 and 2018/19.
A Promising Life Course Approach
The Foresight Programme underpinned by the PHE findings and other public health research prompted a nationwide WCHP based programme of prescribed ‘calorie reduction’ strategies in the general population by 2020. It seems a promising approach, but why do we do this when we know this paradigm may be ineffective? WCHP has been a dominant strategy since the 1980’s, so it appears commonsense to think eating fewer calories will support weight loss, and for all intents and purposes, the model is not without its merits.
By way of the national programme incorporating long-term strategies for improving the food environment. Such as reducing access to calorie-dense foods and beverages by thinning the concentration of fast-food outlets, and aligning the food industry to cut excess calories from major brands, especially those directed at children. Underpinned by levies across the food chain encouraging review and reformulation of products, to lower levels of salt, sugar, and saturated fat calories. Alongside, health awareness campaigns such as Change4Life aimed at addressing the whole family, as well as identifying health inequalities, including obesity stigma.
Importantly, the PHE programme recognises obesity as a chronic disease, in the health system, that predisposes to other diseases, while decreasing overall life expectancy. The WCHP programme under these auspices has shown some level of success when implemented as part of a long-term medical care regime. Protocols that have proven to work include clinical oversight (regular contact with health professionals), oversight from food and nutrition policy including basic nutrition, and physical education, alongside specialist support such as psychotherapeutic or pharmacological interventions, as may be required. Nevertheless, the WCHP conundrum is the shadow of an obesity epidemic. So what else is going on here?
Calorie Reduction Some Science
A review carried out by the National Institute for Health and Care Excellence (NICE, 2013) may begin to shed some light on the challenges faced by weight loss interventions. Following cohorts on national clinical recommended weight loss interventions, they found strong evidence that within 1-year of completing the interventions, the population’s mean weight slowly increased. The average rate of weight regain is estimated as 0.56kg per year. That’s, 5.6 kilograms or 12.35 pounds gained over 10 years based on an ideal daily intake of calories. However, obesity rates tell us the ideal is regularly exceeded.
Researchers have known for decades why WCHP does not work over the long term. Taking for example this very early piece of research is a case in point and harks back to our earlier energy homeostasis discussion. The study was interested in ‘changes in energy expenditure resulting from altered body weight’ and they found that calorie surplus reflected increases in energy expenditure. Conversely, calorie deficits reflected decreases in energy expenditure. A reminder that energy expenditure refers to RMR/ BMR parameters in determining energy balance (accounting for approximately 60% of the total energy expended at rest). Figure 3 shows comparisons of energy expenditure at the end of a period of weight gain and weight loss:
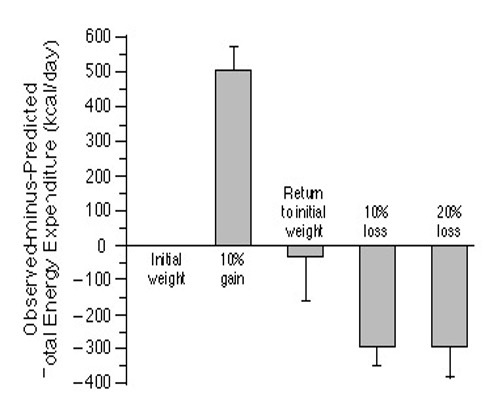
The study found that a 10% increase from usual weight was accompanied by a 12% increase in energy expenditure, suggesting an increased cardiorespiratory load related to an increased number of fat cells (perhaps reflecting hyperphagia or an abnormally increased appetite from raised levels of the hormone leptin). And a 10-20% decrease from the usual weight was accompanied by a 10-15% decrease in energy expenditure, suggesting metabolic resistance to the maintenance of the reduced body weight (possibly underlying are reduced levels of triiodothyronine from thyroxine and raised levels of the hormone insulin). This study has since been replicated many times consistently yielding similar results.
These findings could explain ‘weight plateaus’, and potentially the high prevalence of weight regain, driven by energy homeostasis defending energy balance in returning the body to its so-called setpoint. In obesity where the setpoint is set too high (body fat percentage), then attempts at losing weight will result in adaptive homeostatic controls driving weight regain to return to the setpoint. Another study found that energy deficits predispose homeostatic changes in some people who are lean from weight loss, to accumulate more fat than was lost over time in weight regain and loss of muscle mass, hence the notion posited of ‘fat overshoot’.
Embracing the Principles of Whole-Body Energy Homeostasis
The brain dynamically regulates energy intake and expenditure to meet the continuous energy demands of the brain and body, establishing a theoretical metabolic “set-point”. Investigating the weight set point requires consideration of obesogenic environments and social, economic and cultural factors that may influence various ‘settling points’ in the pursuit of energy balance.
This regulation involves the orchestration of CNS circuits, continuously assessing and integrating metabolic, hormonal, and neuronal signals. The brain adjusts feeding behaviours, hunger, and satiety sensations to respond to acute and chronic energy needs and shortages, ensuring an adequate energy supply for all cell populations.
The brain’s defence mechanisms include adaptations in Resting Metabolic Rate (RMR) or Basal Metabolic Rate (BMR), causing its daily energy expenditure to decline significantly which favours weight gain, altering how the body utilises caloric energy. These homeostatic adaptations represent the brain’s response to uncertainty in a WCHP environment.
Research, especially twin studies, highlights individual differences in food responses based on genetics, and variations in blood sugar and blood fat control. Challenging the WCHP model.
This discussion aimed to shift our ideas about weight loss, moving away from the simplistic WCHP model that concentrates on caloric control and toward a Metabolic-Centred Health Paradigm (MCHP) that concentrates on metabolic regulation.
MCHP offers a comprehensive and adaptable framework for weight management, emphasising whole-body energy homeostasis and understanding of the body’s dynamic regulation of energy. The cornerstone of MCHP is metabolic health research, which depends on the burgeoning and future explorations into ageing science, longevity diets, circadian biology, intermittent fasting and precision nutrition. These endeavours hold significant promise for reshaping our perspectives in this field.
Nevertheless, it is crucial to recognise that scientific viewpoints on these subjects may diverge, and the field is dynamic and subject to continuous research. Emerging findings have the potential to influence and refine our perspectives over time.
Author: Treesje Verlinden
V2.0
This article expresses a new perspective on existing problems, prevalent notions and fundamental concepts relating to existing research and future directions on the topic, and includes personal opinion. It is designed to open up conversation, exploration and debate.o
Aside from a Master’s in related fields of neuroscience do you have any actual titles to give such information to the public? I never heard of a neuroscience professional who can talk about so many topics proving actual expertise. Most of the information you have provided is based on single studies, not systematic reviews, statistics on each statement you made are completely lacking. I mean this respectfully yet with concerns that some of the information provided in actually highly biased. I am an MSc student, and I am a personal trainer qualifying in nutritional strategies. However biostatistics are my main interest in everything I do. This means that unless I see numbers that have actual statistical significance, I simply dismiss whatever the person stated. Unfortunately there is already a much inflated abuse of incomplete biased, partly incorrect, torrent of information polluting our intellectual world.
Excellent post. I definitely appreciate this website. Thanks!